INTRODUCTION
Ovalbumin (54%), ovotransferrin (12%) and ovomucin (3.5%) are major egg white proteins and both their native forms and hydrolysates exhibit remarkable functional properties which can be used as nutraceuticals and pharmaceuticals (Abeyrathne et al., 2013a; Abeyrathne et al., 2018; Mao et al., 2018). Ovalbumin is a glycoprotein with molecular weight of 45 kDa and 386 amino acids (Abeyrathne et al., 2014c) whereas ovotransferrin considered as a monomeric glycoprotein with molecular weight of 76 kDa and 686 amino acids (Abeyrathne et al., 2013b). Ovotransferrin can be separated into apo and holo forms, which significantly differ in their chemical and physical properties whereas, ovomucin is known as a sulfated glycoprotein and the molecular weight is 5.5-8.3 × 103 kDa (Omana et al., 2010; Abeyrathne et al., 2014b).
Nephrolithiasis (kidney stone) is presence of crystal agglomerates caused by a disturbance in the balance between solubility and precipitation of salts in the urinary tract and in the kidney (Han et al., 2015). Approximately 70-80% of kidney stones comprise with calcium oxalate and calcium phosphate. The pathogenesis of calcium oxalate stone formation is a multi-step process, and it includes nucleation, crystal growth, crystal aggregation and crystal retention (Vennila and Ramya, 2016). The supersaturation of urine and low concentration of urinary citrate promote the formation of calcium oxalate crystals (Han et al., 2015).
Urinary oxalate derives from two major sources liver synthesis and the absorption of dietary oxalate (Holmes and Kennedy, 2000). In human, colon is the principal site of oxalate absorption (Prenen et al., 1984). In addition to that, several oxalate specific binding proteins are located in human kidney (Selvam and Kalaiselvi, 2003). These proteins have been identified in human kidney mitochondria and nucleus, intestinal cytosol and intestinal brush border membranes (Adhirai and Selvam, 1998). Moreover, oxalate absorption is influenced by the concentration of other dietary constituents such as calcium, magnesium and fiber (Robertson and Hughes, 1993). Oxalate is a primary chelator in calcium. In plants, oxalate setup as the soluble sodium or potassium salts or as insoluble crystalline calcium oxalate (Naik et al., 2014). The adverse effect of oxalate can be considered in the terms of ratio between oxalate: calcium in a food. Food that has a ratio greater than two, contain no utilizable calcium and have excess oxalate, which can bind calcium in other foods eaten at the same time. Fruits and vegetables such as rhubarb, spinach and beet are good sources of high oxalate content (Noonan and Savage, 1999). Reduction of oxalate intake is a common preventive strategy for calcium oxalate stone formers (Attalla et al., 2014). Because high oxalate and low calcium content lead to hyperoxaluria, kidney stones and kidney failures (Noonan and Savage, 1999).
There are few studies have been investigated to retard the oxalate in diet. Calcium citrate, magnesium citrate administration are the present treatments for idiopathic calcium oxalate kidney stone (Grases et al., 2015). Apart from that, administration of Oxalobacter formigenes is a research level treatment for the formation of calcium oxalate kidney stone (Liebman et al., 2011). According to Savage et al. (2000) oxalate content of the plant tissues could be retard with the heat treatment such as boiling and heating. But these treatments are not always fit for the practical situations like consumption of fresh fruits, fruit juices and curries since most of these methods consist of several problems. Thus, urology specialists are still advising to the patients to reduce their oxalate intake with the diets (Urology specialists of Atlanta, 2018). If egg white proteins use as an oxalate binding agent, it will be beneficial because egg white proteins are non-synthetic natural proteins with many functional and nutraceutical properties.
Accordingly, human and rat kidney contain oxalate binding protein (Selvam and Kannabiran, 1996; Selvam and Kalaiselvi, 2003) and it depends on concentration, pH and temperature (Govindaraj and Selvam, 2002). Furthermore, anion (oxalate) is directly attached to the metal ion in the transferrin protein and oxalate-transferrin complex which is stable at low pH (Halbrooks et al., 2004). After the protein binds with oxalate, the complex (oxalate-protein) will not digest with enzymes (Oke, 1969). Thus, this activity is beneficial to remove oxalate from the body. Ovalbumin, ovomucin, and both holo and apo forms of ovotransferrin are glycoproteins which consists considerably high acidic amino acid sequence than the other proteins except transferrin (Stadelman and Cotterill, 2001). Human and rat kidney contain oxalate binding proteins (Selvam and Kannabiran, 1996; Selvam and Kalaiselvi, 2003) and human serum transferrin has been identified as an oxalate binding protein (Halbrooks et al., 2004). Also, egg white ovotransferrin and peptides or hydrolysates derived from ovalbumin, ovomucin, ovomucoid and ovotransferrin reported to have metal chelating activities (Lin et al., 1994; Ko and Ahn 2008; Abeyrathne et al., 2014c; Abeyrathne et al., 2015; Abeyrathne et al., 2016; Rathnapala et al., 2021). Also, the potential of oxalate binding of these proteins is obviously significant when retard the oxalate amount in the diet. Hence, binding oxalate with egg white proteins and their hydrolysates will be beneficial when retard the oxalate amount in the diet, due to their functional properties and as natural proteins. Therefore, the objective of this study was to determine the oxalate chelating property of ovalbumin, ovotransferrin, ovomucin and their hydrolysates with biogenic oxalate.
MATERIALS AND METHODS
Lyophilized ovalbumin, ovomucin and ovotransferrin which were prepared according to the methods described by Abeyrathne et al. (2014a) were obtained from Iowa State University, Ames, USA. Di sodium oxalate was purchased from Thomas Baker (Mumbai, India). Standard enzymes: protease (form Bacillus licheniformis Alcalase® 2.4L; ≥ 2.4 U/g solution; P4860) and trypsin (from bovine pancreas; ≥ 7500 BAEE U/mg solid; T9201) were purchased from Sigma-Aldrich (St. Louis, MO, USA). Other chemicals were purchased from either from Sigma-Aldrich (St. Louis, MO, USA), Daejung Chemicals and Materials (Siheung, Korea), Research Lab Fine Chem Industries (Mumbai, India) or HiMedia Laboratories (Mumbai, India). Fresh starfruit and spinach were purchased from local market and home gardens of Badulla, Sri Lanka.
Ovalbumin hydrolysate (HOA) was prepared with a combination of 1% (v/v) protease and 1% trypsin (w/v) as described by Abeyrathne et al. (2014c). Both apo-ovotransferrin hydrolysate (HOT-) and holo-ovotransferrin hydrolysate (HOT+) were separately produced using the method of Kim et al. (2012) with trypsin added at 1:25 (v/v) (enzyme: substrate) ratio. Ovomucin hydrolysate (HOM) was selected and produced by incubating at 100°C for 15 minutes as described by Abeyrathne et al. (2016). At the end of each hydrolysis resulting solutions were freeze dried and considered as protein hydrolysates.
Disodium oxalate (Thomas Baker, Mumbai, India) was used to prepare the oxalate samples with slight modification as Naik et al. (2014). Initially, 20 mg/mL oxalate stock solution was prepared and a standard dilution serious was prepared with 5, 10, 15, 20 mg/mL concentrations.
Extraction of biogenic oxalate from spinach (Averrhoa carambola) and starfruit (Spinacia oleracea) was performed according to the protocol of Naik et al. (2014) with some modifications. Fresh materials were washed with running tap water and distilled water and then cut into small pieces separately. After drying at 60°C for overnight, samples were powdered to reduce the particle size. 1.5 g of powdered samples were transferred into 50 mL capacity volumetric flasks and added 30 mL of 0.25 N HCl separately. Then, samples were boiled in boiling water bath for 15 min and cooled into room temperature. Finally, 20 mL of 0.25 N HCl was added to volume up. After the filtration, filtrate was used as the oxalate extract.
First, potential oxalate chelating property of ovalbumin (OA), ovomucin (OM), apo-ovotransferrin (OT-), holo-ovotransferrin (OT+) and their hydrolysates were analyzed with synthetic oxalate. 0.4 g of native proteins or 0.2 g of protein hydrolysates were separately mixed with 10 mL of 20 mg/mL oxalate solution followed by incubating at 4°C for 24 hours. After, samples were centrifuged (Thermo Fisher Scientific-ST 40R centrifuge) at 3,400 rpm, 30°C (facilitate the protein precipitate) for 20 min and supernatant was separated. Secondly, once verified the potentiality of native proteins and their hydrolysates to chelate synthetic oxalate, the biogenic oxalate chelating activity was determined. 0.1 g of proteins and their hydrolysates were added into the 0.4 g of prepared oxalate extracts separately. After incubating at 4°C for 24 hours, samples were centrifuged at 3,400 rpm at 30°C for 20 minutes to separate supernatants.
Supernatants were used to determine the free oxalate using High Performance Liquid Chromatography system (Theromo Fisher Scientific-UltiMate 3000) equipped with diode array detector and with operating conditions as, reverse phase C18 column, methanol: deionized water (50: 50 v/v) for mobile phase, 1 mL/min flow rate and 237 nm of wavelength at 2.593±0.1 min retention time (Suryavanshi et al., 2016).
RESULTS AND DISCUSSION
In this study, all the protein treatments were significantly different (P<0.05) with each other, and OA was reported to have highest chelating activity against synthetic oxalate (64.22±2.28%) and lowest value showed in OT− (25.29±1.19%). In contrast, OT+ exhibited oxalate releasing activity (−47.40±1.81%) (Table 1).
OA | OM | OT− | OT+ | |
---|---|---|---|---|
Native protein | 64.22±2.28%ax | 47.34±2.12%bx | 25.29±1.19%cx | –47.40±1.81%dy |
Protein hydrolysate | 15.10±0.46%ay | 30.33±1.03%ay | 15.40±9.05%ax | 13.63±1.48%ax |
Releasing of oxalate in OT+ is quite deviated from the results obtained by Halbrooks et al. (2004) for human transferrin. As mentioned by Halbrooks et al. (2004), oxalate binds with transferrin groups and the complex are stable in low pH. As an assumption this phenomenon can be describes as, when formation of egg shell, oxalate residuals may already have attached to the ovotransferrin. Interestingly, native OA and OM have shown good oxalate chelation activity.
Furthermore, as shown in Table 1, there was no significant difference of synthetic oxalate chelating activity among all protein hydrolysates. However, HOM exhibited almost doubled synthetic oxalate chelating activity (30.33±1.03%) than other hydrolysates. Moreover, native proteins and their hydrolysates exhibited significantly different synthetic oxalate chelating activity except OT− and HOT−. Native OT+ showed oxalate releasing activity and interestingly HOT+ showed oxalate chelating activity may be due to the effect of hydrolysis. Probably, holo-ovotransferrin which has already bound with Fe3+ did not show oxalate chelating activity. In contrast, showed additional oxalate in the samples. As an assumption, when formation of egg shells these metal-transferrin complexes might be already bound with the oxalate. At the certain conditions these anions may release. Also, as an assumption, the conditions (pH and temperature) might be facilitating the oxalate binding process. When hydrolyzing process, adjusting pH might cause the binding of oxalate. However, Further studies may be essential to prove this phenomenon. However, it is clear there is an effect of hydrolysis on synthetic oxalate chelating activity of HOT+, may be due to exposing new oxalate binding sites in its amino acid sequence.
Extract of spinach contained average 1,234.64±179.18 mg of crude oxalate (data not shown). According to Fig. 1, OA, HOA, HOM, HOT− and HOT+ exhibited more than 85% biogenic oxalate chelating activity (P<0.05) against oxalate extracted from spinach and HOA showed highest activity (94.55±1.22%). Interestingly, all the hydrolysates obtained higher biogenic oxalate chelating activity compared to their native forms and those are having no significant different similar to that of synthetic oxalate chelating activity as described previously in Table 1. When comparing the native forms with their hydrolysates, significantly different biogenic oxalate chelating activity was observed except OA and HOA.
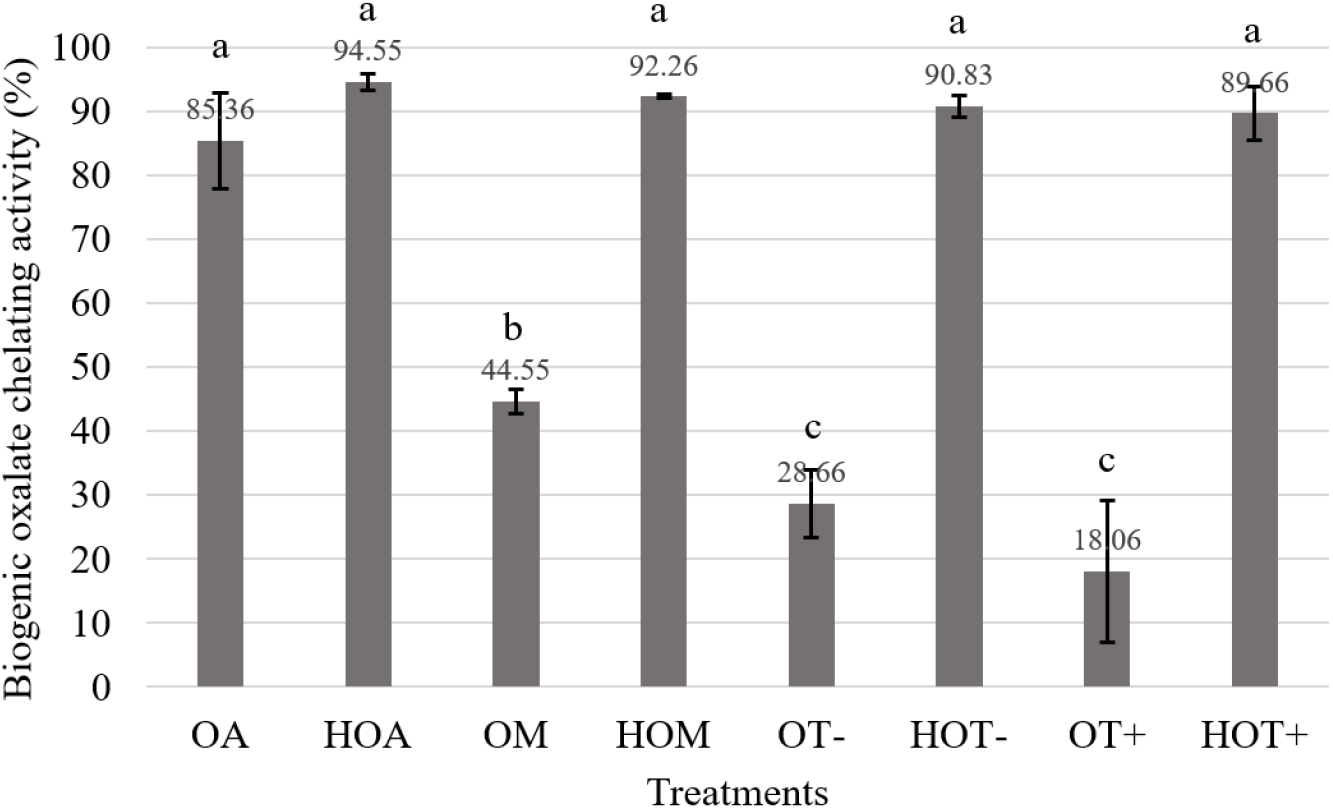
According to Selvem and Kalaiselvi (2003) the attachment of oxalate crystals to the surfaces may depend on the specific composition of surface proteins whether the surface composite with glycolipids or glycoproteins. Thus, it is clear that the carbohydrate moiety is an essential factor for the oxalate binding activity (Fig. 1).
Compared to spinach, starfruit was reported to have low crude oxalate amount; 526.06±126.62 mg (data not shown). Fig 2. shows OA, OM and HOM had more than 50% biogenic oxalate chelating activity (P<0.05). In contrast, other treatments exhibited oxalate releasing activity (P>0.05) which was represented by increasing the amount of oxalate in the media. According to Naik et al. (2014) with the incensement of the acidity, solubility of oxalate was significantly increased. Based on that oxalate, which was bound with native form of protein may release into the extract.
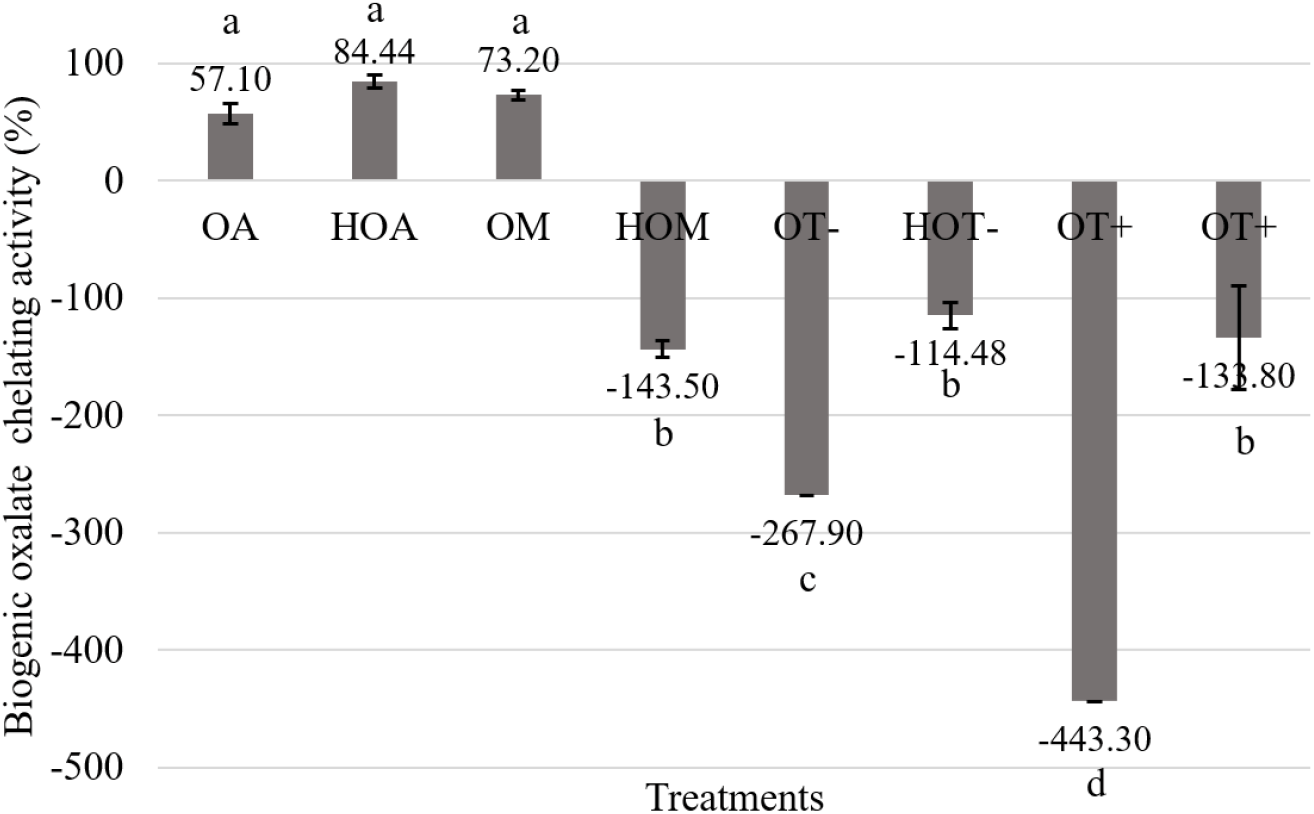
According to Roop-ngam et al. (2012) different forms of albumin and isomers of mucin were identified as oxalate binding proteins in porcine kidney. These proteins facilitated suitable binding surfaces due to their sticking ability. Therefore, it may be affected to getting highest chelation of ovalbumin and ovomucin with biogenic oxalate. Ovomucin consists with the 3 types of carbohydrate chains composed of galactose, galactosamine and sialic acid (Hiidenhovi, 2002). Hence carbohydrate moiety of these proteins facilitates the binding of oxalate. Hence ovalbumin and ovomucin consists with the oxalate binding properties since having both acidic amino acid composition (1/3 of total amino acid composition) and carbohydrate moiety. According to Halbrooks et al. (2004) metal and anion binding ability is a unique feature of transferrin family. Therefore, during the incubation period, oxalate which was bound with ovotransferrin can be released into the extract and the remaining proteins or hydrolysates may bound with released oxalate in starfruit samples. Specially transferrin groups bind with metal ions such as Fe3+ and the binding process has defined by suggesting that the anion (oxalate) is directly attached to the metal ion in the protein. The pH dependence of iron dissociation from iron. Transferrin-oxalate complex is also reported as more stable at low pH than iron-transferrin-carbonate. Apart from that Halbrooks et al. (2004) has showed substitution of oxalate instead of carbonate produce no changes in polypeptide folding in human transferrin.
In this study, investigated all egg white proteins and their hydrolysates showed oxalate chelating activity against oxalate extracted from spinach. However, only few treatments (OA, HOA, OM) showed same activity against oxalate extracted from starfruit and HOM, OT−, HOT−, OT+ and HOT+ exhibited oxalate releasing activity. Even this variation was not studied, factors such as amount of biogenic oxalate present in the spinach and starfruit, purity of extracted biogenic oxalate, physicochemical properties (structure, solubility) of the oxalate and physical conditions such as pH might be affected to the oxalate binding ability.
CONCLUSION
Investigated egg white proteins and their hydrolysates had some biogenic oxalate chelating activity. As per the overall results, HOA was best to chelate biogenic oxalate extracted from both spinach and starfruit. Hence, investigated egg white proteins and hydrolysates have shown emerging pharmaceutical property to develop as an oxalate binding agent. However, further studies are needed to verify the oxalate chelating activity against many plants derived oxalates in both in vitro and in vivo.